Constructing a CBAR Proposal
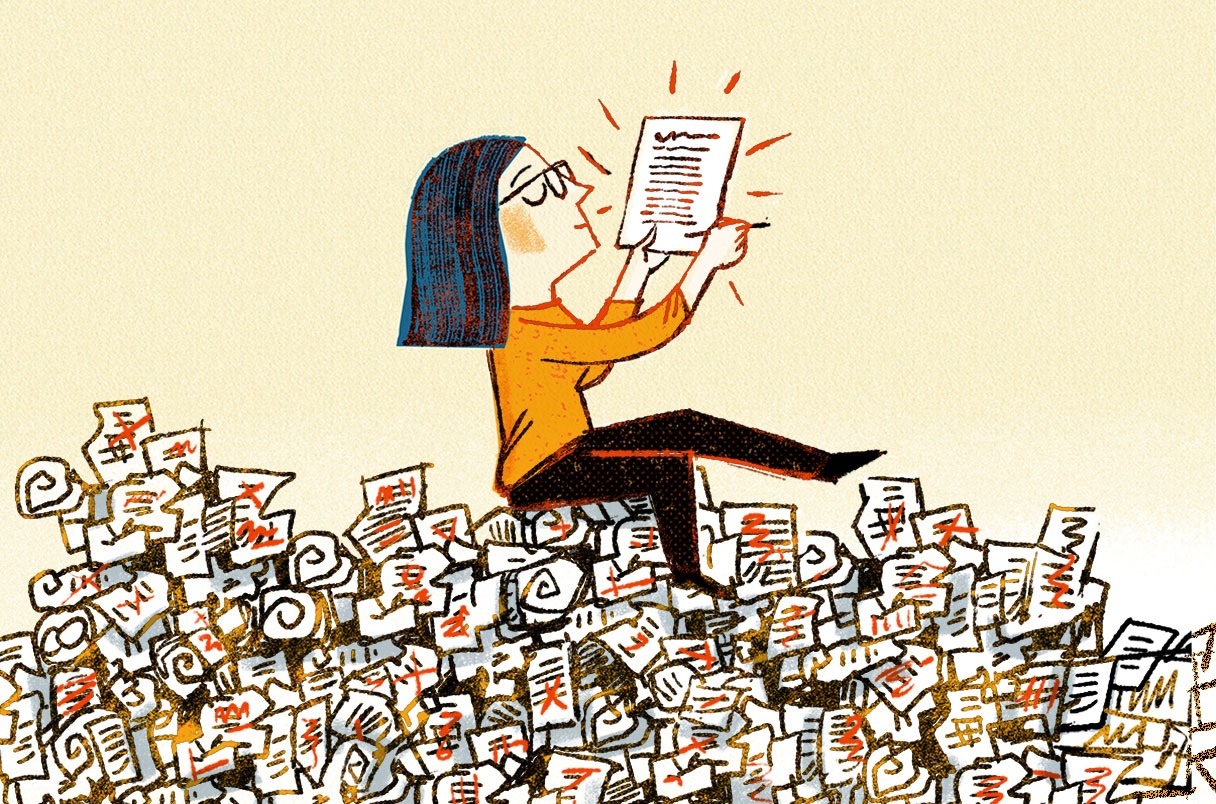
For this task, we were asked to propose our own Classroom-Based Action Research (CBAR) paper. Having developed an understanding of the essential components of an action research report, along with honing our ability to critique research effectively, I felt prepared to draft a concept paper that captures both my experiences and observations in education courses, especially those gleaned from Field Study.
The goal was to create a proposal that not only encapsulates a practical topic but also integrates an actionable intervention based on real classroom needs.
Using Metacognition Instruments to Enhance Introductory Physics Problem-Solving Skills in Multiple-Choice Assessments for Senior High School Grade 11 and 12 Students
Rationale (Brief Description of the study)
This action research examines the effectiveness of metacognitive instruments in enhancing problem-solving skills in introductory physics, particularly through the use of multiple-choice assessments for senior high school students. Metacognition, defined as the ability to monitor and regulate one’s own cognitive processes, has long been recognized as a critical component of effective learning (Avargil et al., 2018; Schraw & Dennison, 1994; Zohar & Barzilai, 2013). In the context of physics education, metacognitive skills are crucial, as they involve being aware of and consciously applying cognitive strategies such as conceptualizing, reasoning, and problem-solving (Veenman, 2012). Despite their importance, many students struggle with these skills when faced with complex physics problems, resulting in difficulties with self-regulation and comprehension (Morphew, 2021; Sundstrom, 2018; Taasoobshirazi et al., 2015).
Physics, as a discipline, requires mastery of abstract concepts and the application of mathematical reasoning (Chabay & Sherwood, 2006). To support students in developing these skills, it is essential to explore how metacognitive strategies can help them better manage their learning processes, reduce misconceptions, and enhance their motivation and self-efficacy (Thomas, 2013; Veenman, 2012). Multiple-choice assessments provide a unique opportunity to test not only factual recall but also deeper understanding of physics concepts, as they challenge students to retrieve and apply knowledge in various contexts (Marsh et al., 2007). Additionally, multiple-choice questions (MCQs) are commonly used in standardized testing due to their objectivity and efficiency in both administration and scoring. Research suggests that MCQs not only enhance retention of knowledge but also support the learning of untested material by encouraging active retrieval (McDaniel et al., 2013; Bjork et al., 2014).
This research aims to investigate how metacognition instruments, when integrated with multiple-choice assessments, can foster improved problem-solving abilities and conceptual understanding in physics education. By focusing on these metacognitive strategies, the study will contribute valuable insights into how students can better navigate complex cognitive tasks in physics, ultimately leading to more effective learning outcomes.
Significance of the study to the research priorities of DepEd / your Institution
The findings of this action research offer valuable insights for various stakeholders in the educational community, particularly in the senior high school department.
For supervisors and principals, the study emphasizes the importance of incorporating metacognitive strategies into curriculum design to enhance students' problem-solving skills in physics. Given the challenges students face with physics in local and international assessments like the Programme for International Student Assessment (PISA), the research shows how reflective practices can make complex concepts more approachable. Strengthening metacognitive skills can improve both classroom performance and standardized test outcomes.
For teachers, this research highlights how metacognitive tools can enhance student performance in multiple-choice assessments, a common format in standardized testing and entrance exams. By fostering self-awareness and strategic thinking, teachers can better prepare Grade 11 and 12 students for these critical exams, leading to improved problem-solving abilities.
Finally, parents can gain an understanding of the importance of metacognitive support in their children's learning process. The research demonstrates how these strategies can help students better manage their study habits, particularly as they prepare for high-stakes entrance exams, leading to improved academic outcomes.
Statement of Purpose/Objectives
The primary aim of this action research is to investigate how metacognitive instruments can be utilized to enhance problem-solving skills in introductory physics, specifically through multiple-choice assessments for senior high school students. The study will examine the effectiveness of metacognitive strategies in helping students regulate their thought processes, improve self-monitoring, and achieve better outcomes in multiple-choice formats, which are commonly used to assess both problem-solving capabilities and conceptual understanding.
The following research questions will guide the study:
- How do metacognitive instruments enhance students' problem-solving skills in multiple-choice assessments in introductory physics?
- To what extent do metacognitive strategies improve students' accuracy and performance in answering physics multiple-choice questions?
- How does the application of metacognitive tools during multiple-choice assessments affect students' ability to self-monitor and regulate their learning?
- What are the common challenges students face when using metacognitive strategies in multiple-choice physics assessments, and how can these challenges be addressed?
While multiple-choice assessments are often favored for their ability to efficiently evaluate large groups of students, their effectiveness in fostering deeper learning depends heavily on the students' ability to engage in reflective thinking. Metacognitive strategies, when employed correctly, offer a structured approach for students to think critically about their problem-solving process. The research will explore how these strategies can be integrated into physics education to help students overcome common misconceptions and increase their confidence in tackling complex problems. However, challenges remain, as some students may find it difficult to adapt to self-regulated learning approaches, which this study aims to address through targeted interventions.
Graphical Conceptual Framework
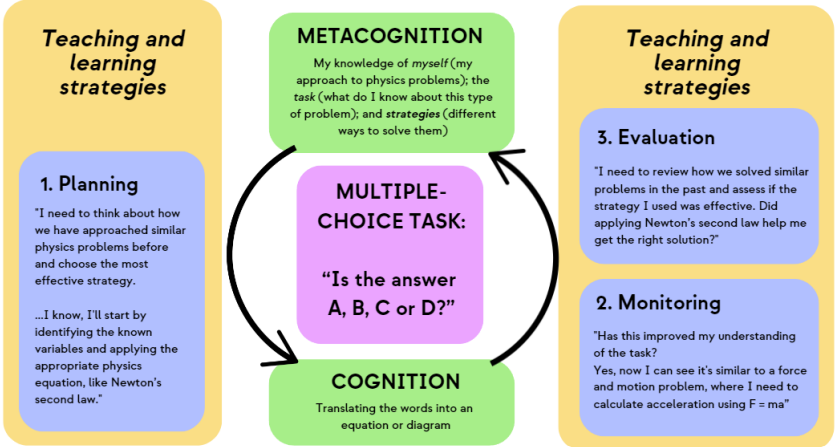
This study builds on the concept of metacognition, which refers to the ability to understand and regulate one’s cognitive processes. Metacognition is commonly described as “thinking about thinking” and is widely recognized as a key factor in academic success. Students who engage in metacognitive practices tend to outperform their peers, especially in subjects that require complex problem-solving skills, such as physics.
This research framework focuses on how teachers can implement the cycle of planning, monitoring, and evaluating to improve physics problem-solving skills among senior high school students during multiple-choice assessments.
The planning phase involves determining what needs to be learned and how to approach the learning process. In the context of physics, this might involve identifying the key principles or laws relevant to a particular problem, such as Newton's laws of motion or the law of conservation of energy. Students must also decide which strategies to employ, such as breaking down the problem into smaller steps, visualizing the physical scenario, or applying the appropriate formulas.
Monitoring requires students to assess their progress throughout the learning process. This involves continually asking questions like, “Do I understand the key concepts?” and “Am I applying the correct formulas and problem-solving techniques?” For physics students, monitoring is crucial as it allows them to identify areas of confusion, evaluate whether their current strategies are effective, and make adjustments if needed.
Evaluation occurs after completing a task, when students reflect on their performance and learning outcomes. In the case of physics problem-solving, this might involve reviewing their answers to multiple-choice questions, analyzing any mistakes, and identifying areas for future improvement. Students may ask questions such as, “Did my approach lead to the correct solution?” or “What can I do differently in the next problem?” Evaluation encourages students to think critically about their learning strategies and make adjustments to enhance their future performance.
Through this framework, students are guided to ask key reflective questions at each stage of their learning process. These reflective questions help them continuously improve their approach to problem-solving, thereby enhancing their overall physics performance.
Methodology
A. Type of Data Needed
The data collection will include a combination of student self-assessment surveys, interviews, and multiple-choice assessment results.
B. Research Instrument for Gathering Data
The self-assessment survey will enable students to evaluate their metacognitive abilities and provide insights into their approaches to problem-solving. This aligns with the idea that metacognitive awareness can be crucial in guiding student learning strategies. Interviews will also be conducted to gain a more comprehensive understanding of students' cognitive processes, self-regulation strategies, and their perspectives on how metacognitive tools have influenced their learning experiences. This qualitative method will allow for a deeper exploration of individual student responses to metacognitive practices, much like research in educational contexts has shown the value of in-depth feedback. Additionally, results from multiple-choice assessments will serve as a quantitative measure of how metacognitive interventions improve both problem-solving skills and conceptual understanding, reflecting approaches used in standardized testing environments to assess cognitive gains.
C. Sample Needed/Respondents
The participants in this study will include senior high school students, who will be divided into three distinct groups: high-performing students, low-performing students, and a control group that meets the expected performance level. Each group will interact with metacognitive instruments in different ways. The control group will follow the standard physics curriculum, without any additional interventions. In contrast, the experimental groups will receive extra support through metacognitive strategies, such as guided reflection and self-monitoring tools, designed to enhance their problem-solving abilities.
This differentiated approach is intended to assess the impact of these strategies on students’ overall performance.
D. Data Collection Procedure
The research procedure will involve a structured approach to assess and improve students' problem-solving skills in physics, using both quantitative and qualitative measures. First, a pre-assessment will be conducted to evaluate students' initial capabilities, focusing on their performance in multiple-choice physics problems. Following this, metacognitive instruments will be introduced to the experimental groups, where students will engage in activities designed to enhance their ability to plan, monitor, and evaluate their problem-solving strategies. Throughout the process, continuous feedback will be collected through self-assessment surveys and interviews to monitor progress. Finally, a post-assessment will be administered using multiple-choice tests, enabling researchers to measure the impact of the metacognitive tools on students' problem-solving abilities.
The seven-step model for teaching metacognitive strategies, as outlined by the Education Endowment Foundation (EEF), offers a more explicit framework for the instructional design of this study. The steps include: (1) Activating prior knowledge, ensuring students connect new learning to what they already know; (2) Explicit strategy instruction, where specific problem-solving techniques are taught; (3) Modelling the strategy, providing students with a clear demonstration of how to apply the techniques; (4) Memorisation of the strategy, ensuring that students can internalize the method; (5) Guided practice, allowing students to apply the strategy with teacher support; (6) Independent practice, where students use the strategy on their own; and (7) Structured reflection, which encourages students to think critically about their learning and performance.
Students may also encounter difficulties in adjusting to metacognitive strategies. Support from teachers and structured guidance will be essential to ensure that students effectively integrate these tools into their learning process.
E. Data Analysis Method
For this study, the quantitative data derived from the multiple-choice assessments will undergo statistical analysis to evaluate performance variations across the three groups. Both descriptive and inferential statistics will be employed to uncover any significant differences in problem-solving abilities. In addition, the qualitative data, collected through self-assessments and interviews, will be analyzed using thematic analysis. This method will help identify patterns in students’ metacognitive awareness, their ability to self-regulate during learning, and their overall engagement with the metacognitive instruments. Thematic analysis is a robust technique for uncovering deeper insights into student experiences.
This mixed-methods approach, combining both quantitative and qualitative data, is expected to provide a comprehensive understanding of how metacognitive tools influence the development of physics problem-solving skills. The integration of both forms of data allows for a broader evaluation, in line with findings from other educational studies that advocate for holistic data interpretation.
Ethical Considerations
In this study, senior high school students will participate, and while they are not considered a vulnerable population, extra care will be taken to ensure their well-being within the educational context. As the students are minors, their participation will require not only their own assent but also parental consent, ensuring that both parties are fully informed and voluntarily agree to participate.
The research environment will be structured to promote a safe, respectful, and non-intrusive atmosphere for all involved. The research poses minimal risk to participants, as it focuses on educational interventions using metacognitive tools and multiple-choice assessments. There are no expected physical risks, and any psychological or emotional risks will be mitigated by carefully monitoring students' experiences. Care will be taken to avoid creating unnecessary stress related to their performance. Confidentiality and data security risks will be addressed through strict adherence to established privacy protocols.
Participants are expected to benefit from engaging with metacognitive strategies that can improve their problem-solving abilities, particularly in physics. These skills may positively impact their overall academic performance. The educational community, including the participating schools, will gain insights into effective methods for enhancing student outcomes in physics and fostering critical thinking. On a broader level, the findings could contribute to improving teaching strategies in physics education.
All data collected during the study will be anonymized to protect the identities of the participants. Personal information will be securely stored, with access limited to the research team. Data, including survey responses, interview recordings, and multiple-choice test results, will be coded for confidentiality. Digital data will be stored in password-protected files, and hard copies will be kept in secure locations. Upon completion of the study, all data will be archived following institutional guidelines and eventually destroyed.
The consent form will include the following components: a clear explanation of the study's purpose, a description of the activities in which participants will engage (such as completing assessments, surveys, and interviews), an outline of potential risks and benefits, a statement on confidentiality and how data will be handled, and a voluntary participation clause affirming that students can withdraw at any time without penalty. The form will also provide contact information for the research team and require signatures from both students and their parents or guardians.
There are no conflicts of interest in this study. The researchers do not have any financial, personal, or professional motivations that could influence the study's results. In the event that any conflicts arise, they will be disclosed to the participants and relevant authorities to maintain transparency. The study is focused on improving educational outcomes, and the results will be shared with the academic community to contribute to better learning practices.
Action Plan
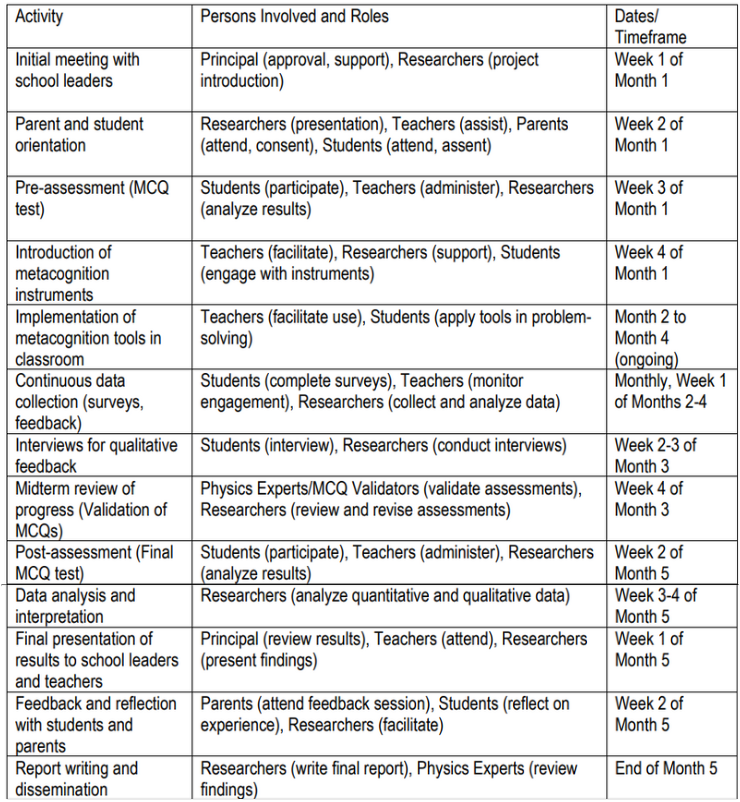
References
Avargil, S., Lavi, R., & Dori, Y. J. (2018). Students’ metacognition and metacognitive strategies in science education. In Cognition, metacognition, and culture in STEM education: Learning, teaching and assessment (pp. 33–64). https://doi.org/10.1007/978-3-319-66659-4_3
Bjork, E. L., Little, J. L., & Storm, B. C. (2014). Multiple-choice testing as a desirable difficulty in the classroom. Journal of Applied Research in Memory and Cognition, 3, 165–170.
Butler, A. C. (2018). Multiple-choice testing in education: Are the best practices for assessment also good for learning? Journal of Applied Research in Memory and Cognition, 7(3), 323–331. https://doi.org/10.1016/j.jarmac.2018.07.002
Chabay, R., & Sherwood, B. (2006). Restructuring the introductory electricity and magnetism course. American Journal of Physics, 74(4), 329–336. https://doi.org/10.1119/1.2165249
Dessie, E., Gebeyehu, D., & Eshetu, F. (2023). Motivation, conceptual understanding, and critical thinking as correlates and predictors of metacognition in introductory physics. Cogent Education, 11(1). https://doi.org/10.1080/2331186X.2023.2290114
Marsh, E. J., Roediger, H. L., III, Bjork, R. A., & Bjork, E. L. (2007). The memorial consequences of multiple-choice testing. Psychonomic Bulletin & Review, 14, 194–199.
McDaniel, M. A., Thomas, R. C., Agarwal, P. K., McDermott, K. B., & Roediger, H. L., III. (2013). Quizzing in middle-school science: Successful transfer performance on classroom exams. Applied Cognitive Psychology, 27, 360–372.
Morphew, J. W. (2021). Changes in metacognitive monitoring accuracy in an introductory physics course. Metacognition and Learning, 16(1), 89–111. https://doi.org/10.1007/s11409-020-09239-3
Schraw, G., & Dennison, R. S. (1994). Assessing metacognitive awareness. Contemporary Educational Psychology, 19(4), 460–475. https://doi.org/10.1006/ceps.1994.1033
Schraw, G., & Moshman, D. (1995). Metacognitive theories. Educational Psychology Review, 7(4), 351–371. https://doi.org/10.1007/BF02212307
Sundstrom, M., Shaffer, P., & White Brahmia, S., & the University of Washington Physics Education Group. (2018). Tracing metacognitive self-regulation in an introductory physics course. https://archive.int.washington.edu/REU/2018/Sundstrom_Paper.pdf
Taasoobshirazi, G., Bailey, M. L., & Farley, J. (2015). Physics metacognition inventory part II: Confirmatory factor analysis and Rasch analysis. International Journal of Science Education, 37(17), 2769–2786. https://doi.org/10.1080/09500693.2015.1104425
Thomas, G. P. (2013). Changing the metacognitive orientation of a classroom environment to stimulate metacognitive reflection regarding the nature of physics learning. International Journal of Science Education, 35(7), 1183–1207. https://doi.org/10.1080/09500693.2013.778438
Veenman, M. V. J. (2012). Metacognition in science education: Definitions, constituents, and their intricate relation with cognition. Contemporary Trends and Issues in Science Education, 40, 21–36. https://doi.org/10.1007/978-94-007-2132-6_2
Post a comment